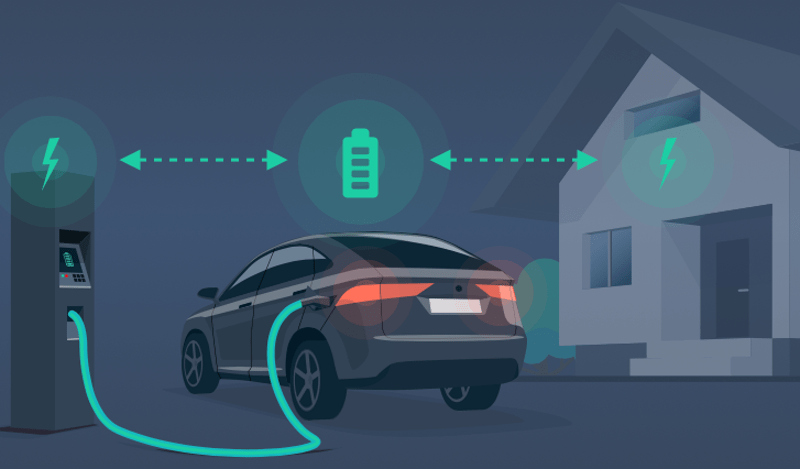
Introduction to Next-Generation Battery Materials
Battery technology has evolved significantly in recent years, playing a pivotal role in various sectors, from consumer electronics to electric vehicles (EVs) and renewable energy storage systems. However, the limitations of traditional battery materials, such as lithium-ion, have prompted researchers to explore next-generation alternatives that promise greater efficiency, longevity, and sustainability.
As the global demand for energy storage solutions continues to rise, driven by the need for green and renewable energy sources, the shortcomings of current battery technologies become increasingly apparent. Challenges such as limited energy density, long charging times, and concerns over resource scarcity and environmental impact necessitate the development of new materials that can power the future more effectively.
Next-generation battery materials represent the forefront of energy storage innovation. These cutting-edge materials are not only set to enhance the performance of batteries but also to address critical issues related to cost, safety, and environmental sustainability. They hold the potential to transform how we store and use energy, making electric vehicles more efficient, mobile devices more durable, and renewable energy storage more reliable.
Researchers are investigating a wide array of materials, including solid-state electrolytes, silicon anodes, lithium-sulfur chemistries, and multi-valent ions, among others. Each of these materials offers unique benefits and could play a significant role in the future of battery technology. The transition to these advanced materials is essential for supporting the growth of emerging technologies and achieving global climate goals.
This blog post embarks on a journey to explore the myriad innovative materials currently under development. By understanding the advantages and potential applications of these next-generation battery components, stakeholders in various industries can better prepare for a future where energy storage is more efficient, resilient, and environmentally friendly.
The Limitations of Current Battery Technologies
Current battery technologies, particularly lithium-ion batteries, dominate the energy storage market due to their widespread use in consumer electronics, electric vehicles, and renewable energy systems. However, despite their prevalence, several critical limitations hinder their performance and broader adoption. One of the primary concerns is the energy density of lithium-ion batteries. They store less energy per unit weight compared to other potential materials, which restricts the operational range of electric vehicles and the effectiveness of portable electronics.
Charge and discharge rates also present significant challenges. Lithium-ion batteries typically require extensive periods to recharge fully, which is impractical for applications necessitating quick turnaround times. In addition, the high discharge rates can lead to heat generation, potentially resulting in battery degradation or safety hazards. These limitations underscore the need for improvements in the efficiency and reliability of energy storage technologies.
The lifespan of lithium-ion batteries is another critical drawback. Over time, these batteries experience capacity loss and reduced efficiency due to repeated charging cycles. This phenomenon, known as cycling degradation, significantly impacts the overall longevity and cost-effectiveness of the technologies that rely on them. As the batteries degrade, they also pose recycling and disposal challenges, adding to their environmental footprint.
Cost is another significant barrier. The production of lithium-ion batteries involves expensive materials and intricate manufacturing processes, which contribute to their high price. This cost constraint restricts the affordability of electric vehicles and other technologies dependent on these batteries, limiting their broader market penetration.
Furthermore, the environmental impact of lithium-ion batteries cannot be overlooked. The extraction and processing of lithium and other materials like cobalt and nickel contribute to environmental degradation and pose ethical concerns regarding labor practices in mining regions. The production and disposal of lithium-ion batteries result in hazardous waste and recycling challenges.
Due to these limitations, there is a significant drive to develop new battery materials and technologies. Innovations aim to enhance energy density, improve charge/discharge rates, extend lifespan, reduce costs, and minimize environmental impact. Addressing these critical issues is essential for the advancement of energy storage solutions, enabling a more sustainable and efficient future.
Solid-State Batteries: A Game Changer
The paradigm shift from traditional liquid electrolyte batteries to solid-state batteries marks a significant advancement in battery technology. Solid-state batteries utilize a solid electrolyte instead of the conventional liquid or gel-based electrolytes, fundamentally altering their structure and operation. This material innovation confers several benefits, propelling the technology into the spotlight of next-generation energy storage solutions.
One of the paramount advantages of solid-state batteries is their higher energy density. The solid electrolytes enable a denser packing of active materials, leading to a more compact yet powerful energy source. This leap in energy density is particularly transformative for electric vehicles (EVs), consumer electronics, and renewable energy systems, where space and weight are critical considerations.
Safety is another cornerstone where solid-state batteries outperform their liquid counterparts. Liquid electrolytes pose risks of leakage, flammability, and thermal runaway, which are significantly mitigated in solid-state designs. The solid electrolyte is more stable and less likely to ignite, thereby enhancing the overall safety profile of these batteries. Additionally, the incorporation of non-flammable materials further reduces the risk of catastrophic failures.
Solid-state batteries also promise a longer lifecycle. The reduced risk of electrolyte degradation and dendrite formation – a common issue in liquid electrolyte batteries that shortens their lifespan – translates into a more durable and reliable energy storage solution. This longevity ensures that devices and vehicles powered by solid-state batteries can operate for extended periods without the need for frequent replacements.
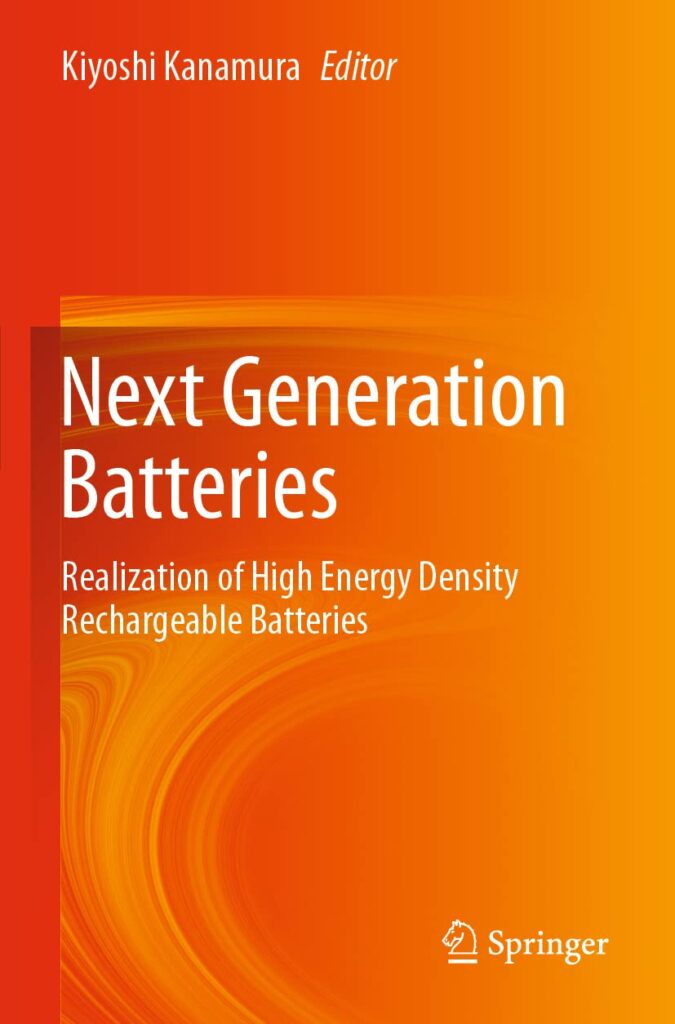
The current research and development landscape for solid-state batteries is vibrant. Numerous academic institutions and private enterprises are investing heavily in overcoming the challenges associated with mass production. This includes resolving issues like high manufacturing costs, scalability of production processes, and ensuring the uniformity and consistency of the solid electrolyte materials.
While promising, solid-state batteries are not without their hurdles. The primary challenges involve ensuring the compatibility of solid electrolytes with existing and emerging battery technologies, mitigating interface resistance, and developing economical manufacturing techniques. Nevertheless, as research progresses and breakthroughs materialize, the potential applications of solid-state batteries span across various sectors including automotive, consumer electronics, and grid storage solutions.
In summary, solid-state batteries represent a pivotal advancement in energy storage technology. With their superior energy density, enhanced safety, and prolonged lifecycle, they are poised to play a crucial role in the future of sustainable energy. Continued research and development will inevitably address the current challenges, heralding a new era in battery technology capable of meeting the growing global demands for clean and efficient energy sources.
Silicon Anodes: Enhancing Lithium-Ion Capacity
The development of next-generation battery materials is a focal point in advancing energy storage technology. Silicon anodes are poised to revolutionize the landscape of lithium-ion batteries, offering a substantial increase in capacity. Unlike traditional graphite anodes, which have been the industry standard, silicon has the theoretical capability to store up to ten times more lithium. This significant increase stems from silicon’s intrinsic properties, particularly its ability to form various lithium silicides, thereby enabling a higher lithium-ion capacity.
However, the implementation of silicon as an anode material is not without its challenges. One of the most pressing technical issues is the substantial volume expansion that silicon undergoes during the lithiation process. This expansion, approximately 300%, can lead to considerable mechanical stress, resulting in particle pulverization and significant capacity degradation over charge-discharge cycles. These mechanical issues have been a major hurdle in the commercial adoption of silicon anodes.
To address these challenges, researchers are exploring innovative approaches, including the development of nanostructured silicon anodes. Nanostructuring silicon can mitigate volume expansion by providing more space for expansion and better accommodation of the strained particles. This strategy involves creating silicon nanowires, nanotubes, and nanoparticles, which can maintain structural integrity and prolong battery life.
Another promising approach is the utilization of composite materials, where silicon is combined with other materials that can buffer its expansion. For instance, incorporating silicon into flexible carbon matrices or binding it with polymers has shown to enhance the cycle life of batteries significantly. These composites can offer a dual function: enhancing electrical conductivity and providing mechanical support to counteract the stress of volume changes.
While silicon anodes present a formidable opportunity to boost the energy density of lithium-ion batteries, overcoming the associated technical barriers is essential for their practical application. Ongoing research and innovation in nanostructuring and composite material design are paving the way towards making high-capacity silicon anodes a viable component in the next generation of energy storage solutions.
The Promise of Lithium-Sulfur Batteries
Lithium-sulfur (Li-S) batteries have emerged as a compelling alternative to traditional lithium-ion batteries, gaining attention due to sulfur’s remarkable properties and advantages. Sulfur boasts an exceptionally high specific energy, which translates to a higher energy density in Li-S batteries compared to their lithium-ion counterparts. This characteristic is pivotal for applications requiring longer battery life and more extended periods between charges, such as electric vehicles and renewable energy storage systems.
From an economic perspective, sulfur is more abundant and considerably less costly than cobalt, which is a critical component in lithium-ion batteries. The abundance of sulfur can potentially mitigate supply chain concerns and lower manufacturing costs, making Li-S batteries a financially viable option for large-scale adoption. This affordability is an exciting prospect for industries aiming to integrate sustainable energy storage solutions.
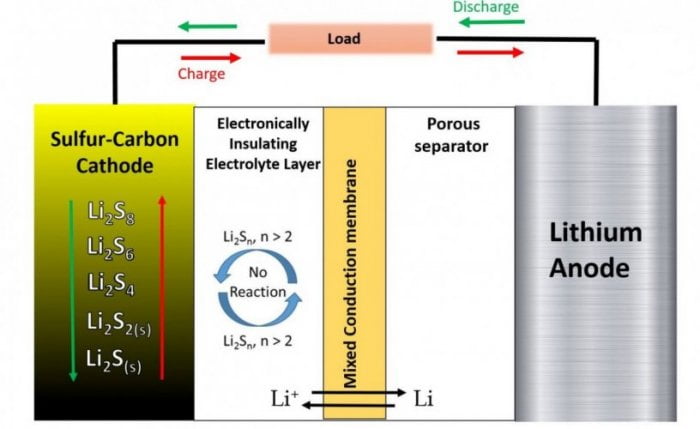
However, the development and commercialization of lithium-sulfur batteries are not without challenges. One significant issue is the ‘shuttle effect,’ where polysulfide intermediates dissolve in the electrolyte during battery operation, leading to reduced efficiency and capacity fading. Another critical challenge is the inherent poor conductivity of sulfur, which impedes its performance as a battery material.
To tackle these hurdles, researchers are pioneering innovative approaches and advanced materials. One such strategy involves the incorporation of carbon-based materials to improve the electrical conductivity of sulfur. These composite materials help in maintaining structural integrity and enhancing electron transport within the battery. Additionally, novel battery architectures, such as the employment of solid-state electrolytes or encapsulation techniques, are being explored to mitigate the shuttle effect and stabilize the battery’s performance.
By addressing these technical obstacles through cutting-edge research and material innovation, lithium-sulfur batteries hold immense promise as a next-generation energy storage solution. The potential benefits, combined with ongoing advancements, place Li-S batteries at the forefront of future developments in energy technology.
Beyond Lithium: Sodium-Ion and Other Emerging Technologies
In the quest for sustainable energy storage solutions, the focus has largely been on lithium-ion batteries. However, innovations such as sodium-ion, zinc-air, and magnesium-ion batteries present a promising frontier. These emerging battery technologies offer distinct advantages and illustrate the potential to address some of the limitations associated with traditional lithium-ion systems.
Sodium-Ion Batteries
Sodium-ion batteries utilize abundant and inexpensive sodium, making them an attractive alternative to lithium-ion systems. Sodium’s abundance ensures supply security and reduces material costs. Moreover, sodium-ion batteries exhibit similar electrochemical performance to lithium-ion batteries at room temperature, making them viable for many applications.
However, sodium-ion batteries are generally heavier and have a lower energy density compared to their lithium counterparts. This limits their use in high-density applications like portable electronics. Nevertheless, they hold substantial potential for grid storage solutions due to their cost-efficiency and resource accessibility.
Zinc-Air Batteries
Known for their high energy density and potential for long-term use, zinc-air batteries utilize zinc and oxygen from the air to generate electricity. These batteries are notably more environmentally friendly, given their reliance on abundant and non-toxic materials. Additionally, the absence of flammable electrolytes makes zinc-air batteries inherently safer.
The main challenge impeding their commercialization is the slow oxygen reduction reaction, which leads to lower power output and efficiency. Research is ongoing to develop more efficient catalysts to enhance reaction rates and overall performance.
Magnesium-Ion Batteries
Magnesium-ion batteries present another emerging technology with significant promise. Magnesium is more abundant and yields higher volumetric energy density compared to lithium. It can also transfer two electrons per ion compared to lithium’s one, theoretically doubling the energy capacity.
Despite these advantages, the technology faces hurdles such as slow ion diffusion rates and compatibility issues with existing electrolytes. Ongoing research aims to overcome these challenges by developing new electrode materials and compatible electrolytes to enhance performance and safety.
Each emerging battery technology has its own set of strengths and weaknesses. While sodium-ion batteries offer cost and resource benefits, they struggle with energy density. Zinc-air batteries promise high energy capacity and safety but face efficiency challenges. Magnesium-ion batteries present high theoretical energy densities but require significant developmental breakthroughs for practical application.
Overall, these emerging technologies highlight the diverse approaches being pursued to improve energy storage solutions. Continued research and development are key to unlocking the full potential of these alternatives and their commercialization in real-world applications.
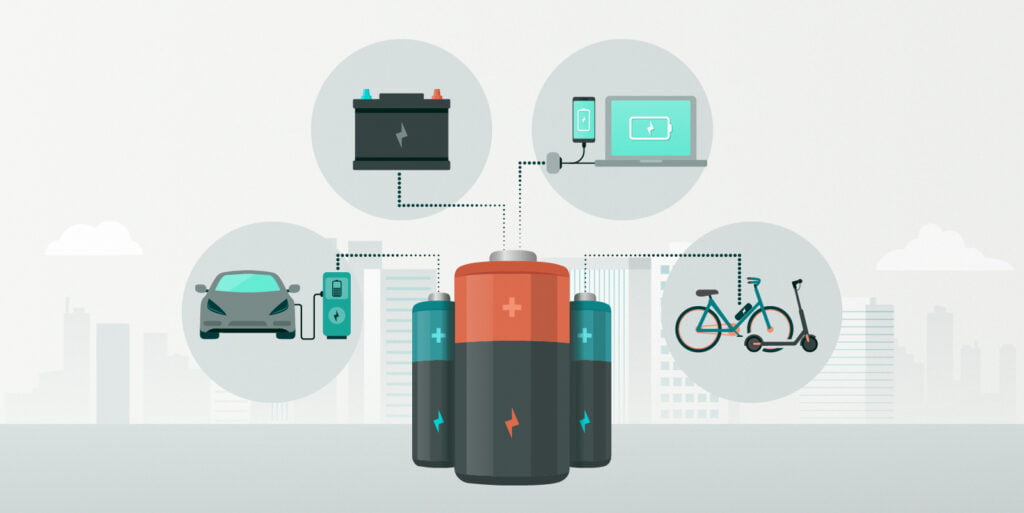
Environmental and Economic Implications of New Battery Materials
The transition to next-generation battery materials carries significant environmental and economic implications, which need careful consideration. One critical aspect is the availability of raw materials. Many advanced batteries rely on elements like lithium, cobalt, and nickel, whose mining and processing pose environmental challenges. For instance, lithium extraction often leads to substantial water consumption, a serious concern in arid regions. To mitigate these issues, researchers and industries are exploring alternative materials and methods that leave a smaller environmental footprint.
Another essential factor is the carbon footprint associated with manufacturing processes. Currently, battery production can be energy-intensive, releasing significant greenhouse gases, particularly if fossil fuels power the manufacturing facilities. Innovations in cleaner production technologies, along with a shift to renewable energy sources, are crucial to reducing the carbon footprint of next-gen batteries. Furthermore, advancing battery efficiency and longevity reduces the demand for frequent replacements, further minimizing environmental impact.
End-of-life recycling and disposal are paramount in understanding the lifecycle implications of new battery technologies. Effective recycling processes can help recover valuable materials, lessen landfill waste, and reduce the need for new raw material extraction. However, achieving efficient recycling systems requires investment in infrastructure and technology, alongside clear policies and regulations. Governments and industry stakeholders must work collaboratively to establish and enforce standards that promote safe and effective recycling practices.
The environmental benefits of these advancements are closely tied to their economic impacts. Sustainable battery materials can spur economic growth by creating new job opportunities in green technology sectors. Moreover, reduced dependence on finite resources and enhanced recycling measures can stabilize supply chains and lower costs over time. However, the initial transition might involve substantial financial investments, which necessitates strategic policy support, such as subsidies and incentives, to encourage adoption.
In essence, the shift to next-generation battery materials offers a promising path towards sustainable energy solutions, but it requires a balanced approach addressing both environmental and economic dimensions. Coordinated effort among scientists, industries, and policymakers is vital to realizing the full potential of these technologies while ensuring an environmentally and economically sustainable future.
The Future of Energy Storage: Trends and Predictions
The landscape of battery technology and energy storage is set for significant transformation in the coming years. As global energy needs continue to escalate, there is an intensified focus on developing more efficient, sustainable, and high-performance batteries. One of the most notable trends is the substantial increase in investment toward research and development (R&D). Governments, private sectors, and academic institutions are dedicating vast resources to uncover breakthroughs in material science that promise to revolutionize energy storage.
Advancements in materials science are particularly critical in this context. One key area of focus is solid-state batteries, which offer higher energy densities, reduced risk of flammability, and faster charging times compared to traditional lithium-ion batteries. Similarly, the exploration of lithium-sulfur and lithium-air batteries could significantly augment energy capacity, while reducing costs. These innovations are not just theoretical but are already moving towards practical applications and commercialization, setting the stage for the next generation of battery materials.
The demand for high-performance batteries is expanding across various sectors such as electric vehicles (EVs), portable electronics, and renewable energy storage systems. The automotive industry, for instance, is rapidly transitioning towards electric propulsion, necessitating batteries that are not only powerful but also cost-effective and long-lasting. Consumer electronics continue to trend towards higher capacity and quicker recharge cycles, driving innovation in battery technology.
In terms of predictive milestones, we can anticipate several key developments within the next decade. The commercialization of solid-state batteries is likely to become mainstream, leading to widespread adoption in EVs and consumer electronics. Breakthroughs in quantum battery technologies might also emerge, promising unprecedented energy efficiencies. Additionally, advancements in battery recycling technologies will play a pivotal role in creating sustainable energy solutions, thereby mitigating the environmental impact of battery disposal.
Looking ahead, these advancements will have a profound impact on everyday life and global energy markets. Enhanced battery technologies will contribute to achieving energy sustainability goals, minimizing carbon footprints, and fostering green innovations across industries. Ultimately, the future of energy storage promises a world where high-performance, reliable, and sustainable battery solutions are the norm, transforming how we harness and utilize energy on a global scale.